Kaiping, China
Seven hundred metres below the rolling green landscape of Kaiping, southeast China, construction workers are furiously finishing a 35-metre-diameter orb-shaped detector that aims to observe ghostly subatomic particles known as neutrinos in exquisite detail. If all goes to plan, the US$376 million Jiangmen Underground Neutrino Observatory (JUNO) will be ready to start detecting by the end of this year, says JUNO’s on-site manager Yuekun Heng, a physicist at the Chinese Academy of Science’s Institute of High Energy Physics in Beijing.
That will make it the first of several ambitious new neutrino detectors currently being built around the world to go online. Two others — in Japan and the United States — are due to start collecting data in 2027 and 2031.
JUNO’s main goal will be to help researchers determine which type of neutrino has the highest mass and which has the least, one of the biggest mysteries in physics. Solving this problem could help physicists to understand what neutrinos are and why their mass is so small. Researchers at JUNO aim to do this by measuring neutrinos pouring in from two nuclear-power stations located more than 50 kilometres away from the observatory. Another goal is to study neutrinos streaming in from other sources, including the Sun, atmosphere, exploding stars and natural radioactive decay processes within the Earth.
On 7 March, researchers at the observatory started to fill a miniature version of the detector with liquid scintillator — a cocktail of solvent and organic chemicals that emits light when neutrinos zip through it. This model will test whether the scintillator is pure enough to help researchers to crack the mass-order problem.
JUNO’s approach sets it apart from the other detectors being built. Japan’s planned Hyper-Kamiokande detector will use purified water as its neutrino-detecting medium, whereas the Deep Underground Neutrino Experiment in the United States will rely on liquid argon to measure the elusive particles, says Mary Bishai, a physicist at the Brookhaven National Laboratory in New York and co-spokesperson for the US observatory. Both of these future detectors will measure neutrinos beaming in from nearby particle accelerators rather than nuclear reactors.
Like telescopes that view the cosmos at different wavelengths, having several neutrino detectors that use distinct techniques to observe neutrinos from various sources, such as the Sun and nuclear power stations, will allow researchers to develop a better understanding of neutrino characteristics and the role of these particles in the Universe, says Bishai. “It gives us a unique way of checking that our picture is consistent,” she says.
The liquid scintillator must contain only minuscule traces of uranium and thorium, radioactive elements that can mimic neutrino events when their decay accidentally coincides with other signals and can destroy experiment results. If levels of these elements are too high, it will be almost impossible to measure neutrinos with the sensitivity needed to solve the mass-ordering problem, says JUNO team member Alberto Garfagnini, a physicist at the University of Padua, Italy. The team is therefore filling the miniature version of JUNO — called OSIRIS — to test the fluid’s radiopurity before it is pumped straight into the main detector next door. It’s important to get this step right, because there’s no going back once JUNO is filled with 20,000 tonnes of the liquid. “It has to be pure from the beginning,” says Garfagnini.
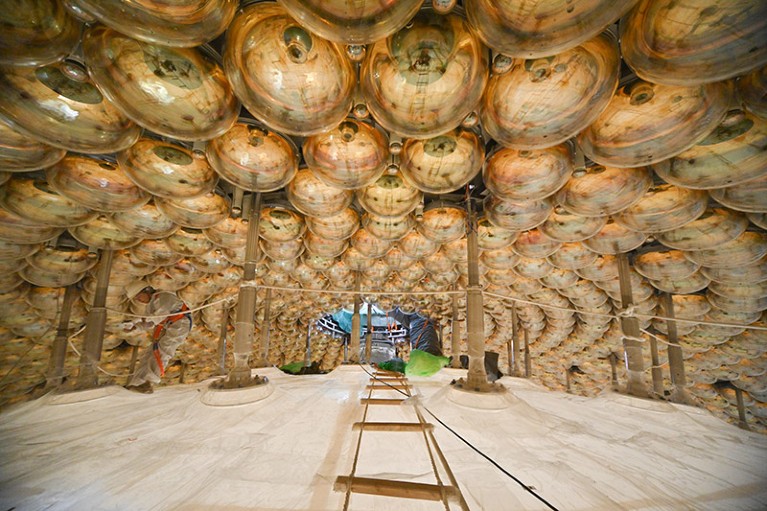
Photomultiplier tubes will detect flashes of energy produced when neutrinos interact with matter.Credit: Institute of High Energy Physics, Chinese Academy of Sciences
Ghostly particles
Table of Contents
Observing a neutrino sounds like it should be easy, given that they are the most abundant particles that have mass in the Universe, with billions of them passing through every cubic centimetre of Earth each second. But their properties remain mostly a mystery, because most of them barely interact with matter while they glide through the cosmos, making it difficult to detect them directly. However, neutrinos might hold clues about how the Universe evolved, says Garfagnini. “They are an important ingredient in cosmology,” he says.
How heavy is a neutrino? Race to weigh mysterious particle heats up
Physicists know that there are three flavours of neutrinos: electron, muon and tau (each named after the fundamental particles they are produced with). More than two decades ago, the Super-Kamiokande experiment in Hida, Japan, and the Sudbury Neutrino Observatory in Canada discovered that neutrinos morph from one flavour into another as they travel1,2, which physicists could explain only if the particles had mass. And in 2012, the Daya Bay Reactor Neutrino Experiment outside Shenzhen, China, precisely measured one of the parameters that describes the rate at which neutrinos switch between flavours3.
Neutrinos also have three mass states — ν1, ν2 and ν3 — and each flavour is a mixture of all of them. Physicists have deduced that ν2 is slightly more massive than ν1, and that there’s a big difference between ν3 and the others. But they still haven’t figured out whether ν3 is heavier or lighter than its better understood counterparts. The answer to this mass-ordering problem has remained elusive, because it demands larger, more-sensitive detectors that are close enough to a well-understood neutrino source, says Bishai. “You have to be in the sweet spot for the effect you are looking for.”
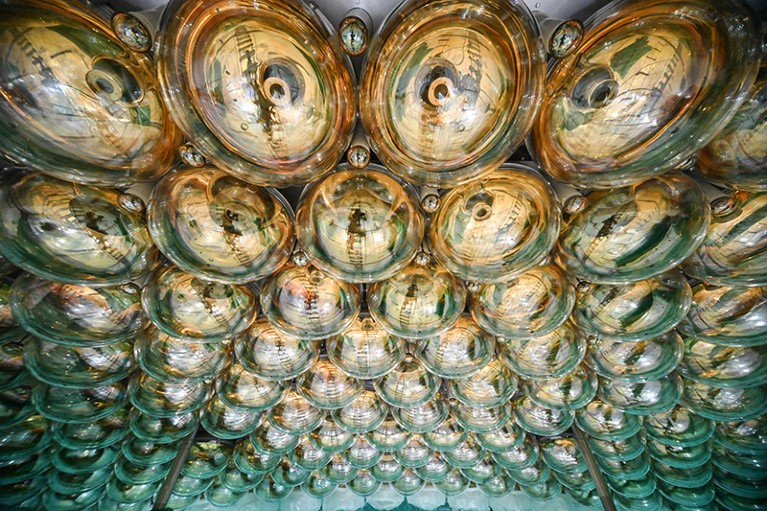
More than 40,000 neutrino-detecting photomultiplier tubes cover the main detector sphere.Credit: Institute of High Energy Physics, Chinese Academy of Sciences
A giant orb
JUNO is located beneath a granite hill, which will act as a shield against cosmic rays — supercharged particles from space that can drown out faint neutrino signals. Every day, fluorescent-vested researchers and construction workers take a 15-minute cable-car ride down a steep 1.3-kilometre tunnel to continue building the detector inside a pristine, temperature-controlled hall. The acrylic sphere, which is roughly two-thirds complete, will soon be submerged in 35,000 tonnes of high-purity water, which will further shield the detector from background radiation. Once the liquid scintillator has passed its radiopurity test, it will be funnelled into the main detector. The entire process will take six months, says Heng.
Safeguarding JUNO’s sensitivity has been no easy feat. When construction started in 2015, the team were hoping to finish the building work in three years. But removing the huge volumes of groundwater resulted in delays. “Water was a big problem,” says Heng. To address this, the team installed a system that pumps 500 cubic metres of water out of the snaking underground tunnels every hour. To control levels of radon — a radioactive gas produced naturally by granite and other rocks that doesn’t play well with sensitive neutrino experiments — the cavernous facility is dotted with whirring, cylinder-shaped fans.
The reason for its difficult location lies on the surface. JUNO sits between two nuclear power stations, each located 53 kilometres away, that will supply the detector with a steady stream of electron antineutrinos, which have the same mass as neutrinos. The sheer number of them churned out by these power plants will give researchers a chance of measuring them with the precision needed to determine their mass order, says Heng.
Neutrinos cannot be detected directly, so to figure out their mass, physicists measure the energy of other particles produced on the rare occasion that a neutrino interacts with matter. In JUNO’s case, when an electron antineutrino bumps into a proton in the liquid scintillator, the interaction will produce a positron and a neutron, a process called inverse beta decay. The energy from the positron results in a flash of light, while the neutron produces another flash when it is captured by a proton. These telltale flashes — 200 microseconds apart — will be measured by more than 40,000 bubble-shaped photomultiplier tubes that will cover the sphere. The time difference between these flashes will help researchers to separate neutrinos from cluttering background signals, says Garfagnini. “It’s a clear signature,” he says. The researchers hope to detect 100,000 neutrinos over the next six years.
Its size, shielded environment and proximity to nuclear power sources will make it one of the most sensitive neutrino detectors in the world, says Geoffrey Taylor, a physicist at the University of Melbourne in Australia. This gives it a good chance of solving the mass order of neutrinos before other experiments get off the ground, he adds. “It’s on track to be a winner.”