The cells glow green under the high-powered microscope, each bedazzled with a constellation of luminous proteins and RNA that, like oil droplets in water, have huddled together through a process known as phase separation.
A foundational concept in the fields of engineering, chemistry and physics, phase separation — the mechanism by which complex mixtures segregate into distinct components — is beginning to revolutionize biology as well. The process is being hailed as a key organizing principle of the cell.
In a fourth-floor laboratory at the Whitehead Institute in Cambridge, Massachusetts, chemical biologist Henry Kilgore has tagged a protein to emit a verdant fluorescent signal wherever throngs of RNA-splicing factors, unbound by membranes, congregate in the cell nucleus to influence gene regulation. “Oh, these look really nice,” he murmurs.
Far from being a disorganized warehouse, the cell more closely resembles an intricate and efficient logistics hub. Clusters of proteins and nucleic acids are compartmentalized into specialized units — called condensates — each with an invisible boundary that is shaped by biochemical affinity and the unique features of phase separation.
The molecules inside are actively sorted, brought together and dispersed, mirroring the bustling efficiency of a dynamic distribution centre. This orderly orchestration underpins the cell’s capacity to regulate its internal environment with precision, allowing for everything from gene control and stress responses to DNA repair and cell division. When this sorting mechanism goes awry, diseases such as cancer, diabetes and neurodegenerative conditions can arise.
Kilgore blasts one condensate with a laser, wiping out its fluorescent signal. Then, he records how quickly the green glow comes back. Called fluorescence recovery after photobleaching, or FRAP, the technique is a popular choice for studying the molecular and fluid dynamics of phase-separated droplets. “This is one of the more convincing demonstrations that a molecule’s mobility can be changed in condensates while doing condensate things,” Kilgore says.
But it’s not the only way to do so. Condensate researchers now have a range of molecular, biophysical and computational tools at their disposal — “technologies that allow us to precisely measure and control phase behaviour in living cells”, says biophysicist Cliff Brangwynne at Princeton University in New Jersey.
A growing number of biotechnology start-up companies — including one that Brangwynne founded, called Nereid Therapeutics in Boston, Massachusetts — now aims to harness these techniques for drug discovery. Academic researchers are playing their part, too. By leveraging these technologies to explore new frontiers in condensate biology, they are transforming the understanding of cellular operations and opening pathways for medical intervention.
“The tools are going to validate themselves,” says Tuomas Knowles, a biophysicist at the University of Cambridge, UK, who doubles as chief executive of Transition Bio in Cambridge, Massachusetts. “These are just incredibly powerful techniques, and they are going to really drive this field forward.”
Suspended belief
Table of Contents
The pioneering US cell biologist Edmund Beecher Wilson had none of these tools when, in 1899, he conjectured1, on the basis of crude imaging of sea-star eggs, that cells might harbour “a mixture of liquids” interspersed with “suspended drops … of different chemical nature”.
Yet, it would take more than a century for scientists to prove Wilson right.
The shape-shifting blobs that shook up cell biology
In 2008, at a summer workshop in Woods Hole, Massachusetts, a team led by Brangwynne and his postdoctoral adviser, cell biologist Tony Hyman at the Max Planck Institute of Molecular Cell Biology and Genetics in Dresden, Germany, noticed that clusters of RNA and protein found in worm embryos behave like liquids, even though they were thought to be solid2.
Like oil droplets in a well-mixed vinaigrette, the structures seemed to coalesce and dissolve, choreographed by some kind of intrinsic molecular or biophysical pull.
“It was surprising and cool,” says Brangwynne, who, together with Hyman, was awarded the 2023 Breakthrough Prize in Life Sciences, in part for this work.
Researchers around the world jumped on the discovery, delineating condensates in their own model systems and seeking the rules that govern their assembly. Many condensates seemed to be built around disordered proteins, which, although lacking a rigid structure, have the flexibility to interact with other molecules in versatile and dynamic ways.
Scientists can visualize these structures by tagging proteins with light-emitting markers — as Kilgore did with his splicing-factor protein — and tracking where the glowing signal aggregates in cells. But that method only works for naturally occurring condensates. And as Knowles points out: “You can’t really understand something unless you can take it apart and control it.”
A major advance, therefore, has been the ability to toggle condensate formation at will, a capability that became possible after the introduction of light-tunable technologies with playful names such as optoDroplet3 and OptoGranules4.
These ‘optogenetic’ platforms take advantage of special light-responsive domains that are fused onto condensate-prone proteins of interest. When exposed to a specific wavelength of light, these engineered proteins self-aggregate. This triggers condensate assembly and provides a powerful tool for researchers to observe and analyse the process in real time.
Academic scientists have embraced these tools, and used them to dissect the material properties of condensates and the molecular interactions that propel phase separation. “And as time goes by, we’ll be able to design these tools more carefully such that we can interrogate the real driving forces underlying endogenous condensates,” says Dan Bracha, a bioengineer at the Technion—Israel Institute of Technology in Haifa.
At Nereid, scientists are leveraging Corelet, an optogenetic technology co-developed5 by Bracha during his postdoctoral tenure in Brangwynne’s lab, to initiate condensate formation in cellular models. The technology is key to the firm’s strategy of identifying therapeutic compounds that can alter the dynamics of phase separation, says Nereid chief scientific officer John Reilly.
For neurodegenerative disease, in which abnormal condensate formation is implicated, the company’s objective is to find molecules that can prevent these condensates from assembling. Conversely, for scenarios in which condensates are beneficial, such as in enhancing the expression of genes that fight tumours, Nereid focuses on compounds that promote phase separation.
“It’s a great screening tool,” Reilly says. “It has given us actionable small molecules that we can now turn into drugs.”
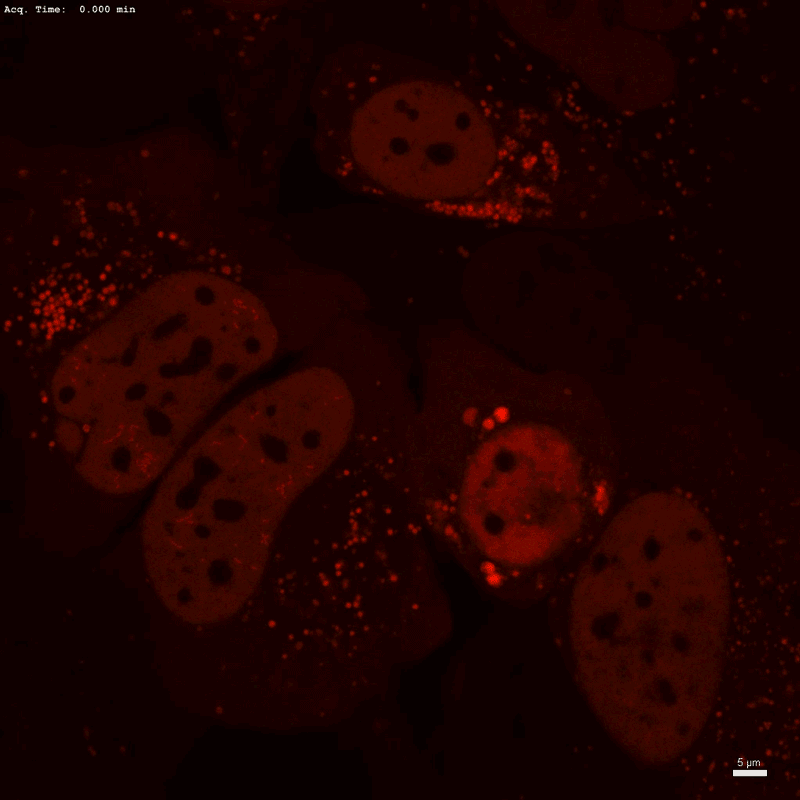
Optogenetic technology Corelet initiates condensate formation in the nucleus. Phase separation occurs after activation with light.Credit: Dan Bracha and Cliff Brangwynne
But optogenetic systems like Corelet only work with proteins that are already known to form condensates. That’s not a problem for drug hunters such as Reilly, who want to perturb condensate dynamics in natural settings for therapeutic gain. But many synthetic biologists are also seeking to harness condensate-mediated organization to imbue cells with new and interesting capabilities, such as enhanced drug production or the formation of super-crops and microorganisms.
“We want to build simple, engineerable, modular systems,” says Ashutosh Chilkoti, a biomedical engineer at Duke University in Durham, North Carolina.
Under control
In 2023, Chilkoti unveiled a series of artificial proteins that provide this level of control over condensate formation in bacterial cells. Guided by the molecular principles of phase separation, he and his colleagues — including Duke synthetic biologist Lingchong You and chemical biologist Yifan Dai, who is now at Washington University in St. Louis, Missouri — crafted shape-shifting proteins with repeating peptide sequences. These not only mimic the structure of disordered proteins found in natural condensates, but can also be fine-tuned to enable precise command over what they do.
Some of the bespoke condensates the researchers made boosted gene expression, whereas others effectively isolated target proteins from degradation components, extending their half-life6. A few even modified the distribution of charged particles in the cell, leading to electrochemical shifts that influenced cellular stress responses and overall gene-activity patterns7. “It’s programmable in a way that you can have precise regulation,” explains You.
What lava lamps and vinaigrette can teach us about cell biology
The team behind these configurable condensates is mostly looking to harness the structures for synthetic-biology applications. But, as Dai points out, the same tools are also revealing the biochemical features that govern phase separation inside cells and suggesting never-before-seen functions for condensates — for example, creating pH gradients and electrical potentials — that others can now test. “It’s generating new hypotheses,” he says.
Similarly, biochemist Dek Woolfson and his colleagues at the University of Bristol, UK, have crafted synthetic proteins that are capable of co-condensing with one another. Each protein in the researchers’ design gets strategically fused to different enzymes that act in tandem to carry out a common biochemical function. Phase separation brings these proteins together, enhancing the efficiency of the associated pathway.
As a proof of concept, the researchers engineered condensates that would co-localize a pair of enzymes involved in the two-step conversion of the amino acid tryptophan to indigo, a blue dye. Bacteria expressing these designer condensates produced up to six times more indigo compared with bacteria with free-floating enzymes dispersed separately throughout the cell8.
According to co-author Alex Hilditch, a former doctoral student in Woolfson’s lab who is now at the Swiss Federal Institute of Technology in Lausanne (EPFL), this synthetic co-condensation strategy has the potential to optimize a wide range of enzymatic processes in bacterial-cell manufacturing. “And the real benefit,” he says, “is that, because you are just co-localizing things” — rather than altering their expression levels — “hopefully you shouldn’t increase the metabolic burden on your chassis organism”.
Fit for repurpose
Even with these newer technologies, the mainstays of condensate research remain older methods such as FRAP that have been adapted for phase separation but originated in other fields of enquiry. “There’s a lot of repurposing,” says Sua Myong, a molecular biophysicist at Boston Children’s Hospital. And a lot of poking, prodding, and mixing and matching of different techniques, often in both test tubes and living cells.
“Every procedure has its own caveats,” explains Alessandra Dall’Agnese, a cell biologist at the Whitehead. “This is why it is important to accumulate orthogonal lines of evidence that support, or not, your hypothesis.”
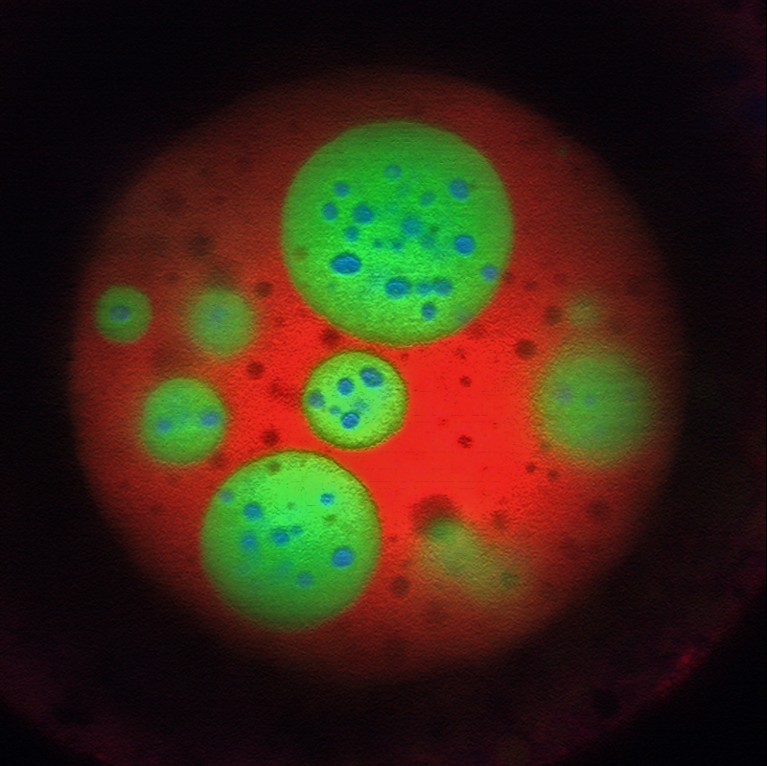
Phase separation inside the nucleolus.Credit: Marina Feric and Cliff Brangwynne
For molecular imaging, for example, researchers often tag proteins of interest with fluorescent markers. But these biochemical dongles can change the solubility or charge distribution of a protein, altering the kinetics of phase separation. “You have to be mindful of picking the right fluorescent protein to minimize these confounding effects,” says Jonathon Ditlev, a cellular biophysicist at the Hospital for Sick Children in Toronto, Canada.
Yet another consideration is that many of today’s laboratory techniques only work with big condensates — those that either can be seen under a microscope, or that contain artificially high concentrations of their constituent parts. Condensates come in a range of sizes, however. And it is quite possible, says Steph Weber, a cell biologist at McGill University in Montreal, Canada, that condensation inside small droplets happens by a completely different means, with different functional consequences, than it does for visible conglomerates.
“We’ve been looking at all these big things,” Weber says. But what happens inside smaller clusters could be equally, or more, important determinants of cellular order. “That might be where the action is.”
From condensates to compounds
Translating any experimental insight into actionable treatments is a complex task — a challenge well known to the team at one-time biomolecular firm Faze Medicines.
Company scientists had been trying to develop small molecules that could rectify the aberrant phase changes associated with cancer and motor neuron disease (also known as amyotrophic lateral sclerosis). They designed high-throughput assays to identify drug candidates that are capable of disrupting harmful interactions in condensates in a controlled laboratory setting9. Promising compounds could then be advanced to testing in living cells.
But, as Rachel Meyers, the company’s former chief scientific officer, points out: “It’s complicated biology.” And when broader economic pressures led investors to consolidate their holdings, the company was forced to cease operations. “We just weren’t far enough along,” Meyers says.
NatureTech hub
One company that is further along is Dewpoint Therapeutics in Boston. Last year, the firm, which was co-founded by Hyman and Whitehead biologist Rick Young (Dall’Agnese and Kilgore are both members of Young’s lab and consult for Dewpoint), unveiled a series of condensate-modifying drugs capable of rescuing motor neurons from the ravages of motor neuron disease, at least in cell culture. When administered at therapeutically relevant concentrations, these drugs helped to restore gene activity to normal levels and reduced the harmful effects of stress on the neurons, specifically preventing the cellular extensions known as axons and dendrites from shrinking, a common issue in the disease.
The company found these molecules through a phenotypic screening strategy, using robotic systems to bombard cells with an array of 370,000 unique molecules from Dewpoint’s chemical library. The process uses automated imaging systems and advanced artificial intelligence (AI) software to capture, quantify and sort through high-resolution pictures of the cells, looking for changes in the size, position or composition of condensates that could signal potential therapeutic benefits.
“It’s a discovery and technology platform,” explains chief scientific officer Isaac Klein — one that allows for “precision alteration of condensate behaviour”.
AI also underpins the drug-discovery philosophy at Transition Bio, which has branded its research platform Condensomics. The company uses a microfluidic system developed in Knowles’s academic laboratory for studying condensate dynamics under tens of thousands of conditions10. Data are then fed into machine-learning algorithms designed to tease apart the molecular ‘grammar’ of phase separation, aiding target prediction and drug design11.
“There’s enormous potential to include these techniques now in almost all aspects of our work,” says Knowles, who last year described a series of antimicrobial peptides discovered in this way that phase separate together with nucleic acids inside bacteria to exert their inhibitory effects12. “If you want to understand the sequence grammar,” he adds, “AI is really the only way to do that.”
Knowles’s AI model, termed DeePhase, forecasts protein entry into condensates on the basis of their structural pliability, fostering interactions at disordered regions that lead to phase separation. Yet, as Kilgore points out, these regions are not the only drivers of phase separation; other structures play a part as well.
Together with members of Regina Barzilay’s computer science lab at the Massachusetts Institute of Technology in Cambridge, Kilgore has been developing an AI tool that takes a holistic view of proteins and can even suggest sequences that should undergo phase separation inside cells. By integrating machine learning with these kinds of AI-generated sequence, Kilgore foresees a deeper understanding of the fundamental mechanisms and chemical principles that govern these enigmatic cellular structures, ushering in a new era of discovery for academics and drug developers alike.
Back in his laboratory, Kilgore zaps another condensate. As he considers the technical toolbox slowly taking shape in his field, he muses aloud: “It’s really going to be a game changer.”